[ad_1]
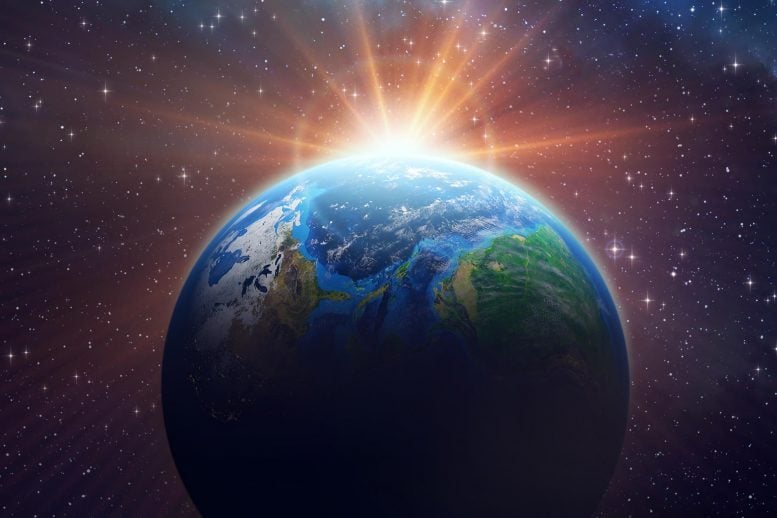
Through high-energy laser experiments, the researchers revealed that magnesium oxide is likely to be the first mineral to solidify during the formation of super-Earths, with crucial implications for the geophysical evolution of these planets.
A new study reveals that magnesium oxide, a key mineral in planetary formation, may solidify first on developing “super-Earth” exoplanets, and its behavior under extreme conditions could have major implications for planetary development.
Scientists have observed for the first time how magnesium oxide atoms deform and dissolve under extremely harsh conditions, providing new insights into this important mineral in Earth’s mantle that is known to influence planetary formation.
High-energy laser experiments exposed tiny crystals of this mineral to heat and pressure similar to those found deep within the mantle of a rocky planet, suggesting that the compound may be the oldest mineral to have solidified from a magma ocean during the formation of a “super-Earth” exoplanet.
“Magnesium oxide may be the most important solid controlling the thermodynamics of young super-Earths,” said June Wicks, assistant professor of Earth and Planetary Sciences at Johns Hopkins University, who led the study. “With its extremely high melting point, it would be the first solid to crystallize as a hot, rocky planet begins to cool and its interior separates into a core and mantle.”
Impact on young planets
The results of this study are: Scientific advances.
The team suggests that the process by which magnesium oxide changes from one form to another could have important effects on the factors that control whether young planets turn into snowballs or lava, develop water oceans or atmospheres, or a mix of these characteristics.
“In Terrestrial super-Earths, this material is a large component of the mantle, so changes in it will have a major impact on the rate of heat transfer in the interior, which in turn will control how the interior and the rest of the planet form and deform over time,” Wicks said. “You can think of this as a proxy for the interiors of these planets, because this material is the material that controls deformation and is one of the most important components of rocky planets.”
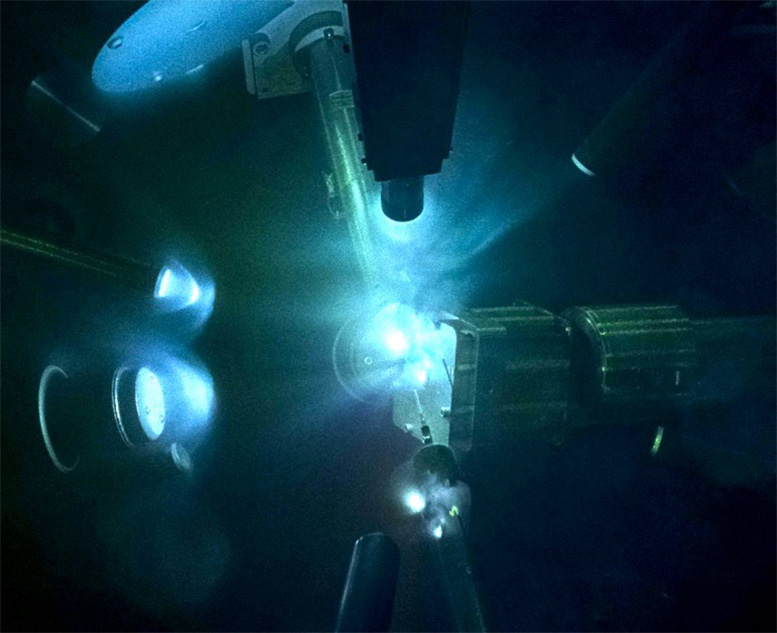
A view of the laser-driven experiment of shock-compressing magnesium oxide (MgO) inside a chamber at the Laser Energetics Laboratory. A high-powered laser beam is used to compress MgO samples to pressures exceeding those at the center of the Earth. A secondary X-ray source is used to probe the crystal structure of MgO. The bright areas are plasma emissions that glow on nanosecond timescales. Credit: June Wicks/Johns Hopkins University
It is bigger than the Earth, Neptune or UranusSuper Earths are Exoplanets These planets have been the subject of exploration because they are common in other solar systems in the galaxy. The compositions of these planets range from gas to ice to water, but rocky super-Earths are expected to contain large amounts of magnesium oxide, which, like Earth, could affect the planet’s magnetic field, volcanism and other important geophysics, Wicks said.
To recreate the extreme conditions the mineral would endure during planet formation, Wick’s team subjected small samples to extreme pressures using the Omega EP laser facility at the University of Rochester’s Institute for Laser Energetics. The scientists also shot X-rays at the crystals and recorded how the beams bounced off of them, tracking how the crystals’ atoms rearranged in response to increased pressure, particularly when they changed from a solid to a liquid.
When compressed very hard, the atoms of materials like magnesium oxide rearrange to better withstand the compressive pressure, so as the pressure increases, minerals change from a halite “phase,” similar to table salt, to a different configuration, like another salt called cesium chloride. This change could affect the viscosity of the minerals and their effect on the planet as they grow, Wicks said.
Magnesium oxide stability under high pressure.
The team’s results show that magnesium oxide can exist in either phase at pressures of 430-500 gigapascals and temperatures of 9,700 Kelvin – nearly twice that at the surface of the sun. The experiments also show that the maximum pressure the mineral can withstand before completely melting is more than 600 gigapascals, roughly 600 times the pressure felt at the deepest part of the ocean trench.
“Magnesium oxide melts at a much higher temperature than other materials and minerals. Diamond may be the hardest material, but it is the last to melt,” Wicks said. “When it comes to extreme materials on young planets, magnesium oxide is likely to be solid, but all the other materials floating below the mantle will turn into liquids.”
The study demonstrates the stability and simplicity of magnesium oxide under extreme pressure, and could help scientists develop more accurate theoretical models to explore key questions about the behavior of this material and other minerals on rocky planets like Earth, Wicks said.
“This study is a love letter to magnesium oxide, because it’s amazing that at pressures exceeding those at the center of the Earth, it has the highest melting point we know of, yet behaves like a normal salt,” Wicks said. “It’s just a beautiful, simple salt, even at record pressures and temperatures.”
Reference: “B1-B2 transition in shock-compressed MgO”, June K. Wicks, Saransh Singh, Marius Millot, Dayne E. Fratanduono, Federica Coppari, Martin G. Gorman, Zixuan Ye, J. Ryan Rygg, Anirudh Hari, Jon H. Eggert, Thomas S. Duffy, Raymond F. Smith, 7 June 2024, Scientific advances.
Source: sciadv.adk0306
Other authors include Saransh Singh, Marius Millot, Dayne E. Fratanduono, Federica Coppari, Martin G. Gorman, Jon H. Eggert, and Raymond F. Smith of Lawrence Livermore National Laboratory; Zixuan Ye and Anirudh Hari of Johns Hopkins University; J. Ryan Rygg of the University of Rochester; and Princeton University.
This research was supported through the NNSA’s National Laser User Facility Program (Contract Nos. DE-NA0002154 and DE-NA0002720) and LLNL’s Laboratory Initiated Research and Development Program (Project No. 15-ERD-012). This work was carried out by Lawrence Livermore National Laboratory under Contract No. DE-AC52-07NA27344 with support from the U.S. Department of Energy. This research was supported by the National Nuclear Security Administration through the National Laser User Facility Program (Contract Nos. DE-NA0002154 and DE-NA0002720) and LLNL’s Laboratory Initiated Research and Development Program (Project Nos. 15-ERD-014, 17-ERD-014, and 20-ERD-044).
[ad_2]
Source link